Quantum Metrology
Metrology – the science of measurement – strives to continually create ever more accurate and precise measurements. However, quantum mechanics sets fundamental limits on the total amount of information it is possible to know about a system. For example, the Heisenberg uncertainty principle sets restrictions on the theoretical measurement uncertainty on a pair of conjugate variables. In addition, as we measure more precisely the discrete and probabilistic nature of reality imposes constraints on the scope of measurements. Quantum metrology looks to push the precision of our measurement devices beyond these standard limits.
1949: First Atomic Clock
Traditional clocks relying on the natural oscillation frequency of quartz crystals are highly dependent on temperature, accruing significant errors. A search for more accurate devices led to the concept of timekeeping by measuring the frequency of atomic transitions, a highly stable parameter. NIST developed the first atomic clock using vibrations of molecular ammonia, with its derivatives used in GPS satellites.
Reference: A review by NIST looks back on their first 100 years of time and frequency measurement research. This stretches back to the first atomic clock in 1949. https://doi.org/10.1109/FREQ.2001.956152
This website details NISTs work on atomic clocks.
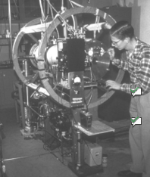
1963: First Josephson Junction
Josephson junctions were experimentally realised by Anderson et al. These quantum devices are macroscopic superconducting junctions and would form the basis of superconducting qubits.
Reference: https://doi.org/10.1103/PhysRevLett.10.230
An introduction to Josephson Junctions, with their associated use in SQUID’s for magnetic sensors.
1964: First SQUID
SQUID’s, superconducting quantum interference devices, were first invented by Jaklevic et al. by placing two Josephson junctions on a closed loop. SQUID magnetometers are highly sensitive and are capable of detecting a single magnetic flux quantum.
Reference: This paper describes the first measurements of a direct current SQUID magnetometer, building on the platform of the first Josephson junction a year prior. https://doi.org/10.1103/PhysRevLett.12.159
This review paper provides an overview of the principles of a SQUID in addition to a qualitative description of their manufacture and operation.
1985: First Squeezed Light
Slusher et al. generated squeezed light for the first time by harnessing the non-linear process of four wave mixing. Due to the Heisenberg uncertainty principle, it is impossible to know two conjugate variables precisely. By squeezing the light, the uncertainty in one of these can be decreased at the expense of increasing the uncertainty of the other variable. They reduced the noise fluctuation in one of the variables of the light below the shot noise limit - the limit of precision placed on classical light. Techniques like this would go on to form the basis of ultra-sensitive quantum measurements with applications in metrology and computing.
Reference: https://doi.org/10.1103/PhysRevLett.55.2409
This perspective introduces the formalism behind squeezing of light in an approachable and intuitive fashion. It then focuses on the applicability to enhanced sensing and metrology enabled by this process. The work also offers a perspective on the possibility of order of magnitude future improvements.
Above: A diagram demonstrating the effect of squeezing light. Light can be represented using its Position (X) and Momentum (P) on a set of axes with the distance from the centre related to the number of photons in the state of the light the size of the blob showing the uncertainty in in this position and momentum. By squeezing the light, we see the size of the blob reducing in one direction and increasing in the perpendicular direction, indicating a change in uncertainty. Commonly we squeeze along either the position or momentum axis. However, as the uncertainty principle cannot be violated the product of the uncertainty along these axes cannot be below h/2.
1991: Atom Interferometry
Within an optical interferometer light is split into two independent arms, before being recombined. Any phase difference acquired between the arms leads to an interference pattern. Within the proposed atom interferometer light is replaced by atoms, utilising the wave-like behaviour of matter. An individual atom is put in a superposition of states which are then specially separated into two arms and manipulated using laser pulses. A phase change acquired between the arms creates an interference pattern which is detected when the atomic state is measured. This technique can be employed to create high precision measurement devices.
Reference: https://doi.org/10.1103/PhysRevLett.67.181
An easy-to-understand introduction to atom interferometry by Professor Pritchard provides context to matter interferometry starting from optical interference. He touches on his work on polarizability measurements using these concepts, as well as inertial measurements.
Above: An image of a commercially available atom interferometer. These are similar to optical interferometers but use ultra-cold atoms to make high precision measurements using atoms in superposition which are separated and then manipulated using lasers. One of the arms acquires a phase difference which can be measured to an extremely high precision. Atom interferometers are often used to make very sensitive measurements of variations in gravity (gravimeters) but can also be used to measure acceleration (accelerometer) and as rotation sensors.
1998: Quantum Gravimeter
A design for an atomic interferometer used to measure acceleration due to gravity was proposed, with a theoretical precision higher than the best available classical gravimeters.
Reference: https://doi.org/10.1088/0026-1394%2F38%2F1%2F4
This video by Stanford Professor Kasevich looks at a test of quantum mechanics and gravity with atom interferometry. He highlights recent advances in the distance over which interference can be maintained and the resulting impact on the measurement sensitivity.
2014: Imaging without detection
A protocol was proposed to detect objects by measuring exclusively photons which do not interact with the object itself. This uses entangled photon states which interfere such that the single photon counts reveal the object’s transmittance and phase profile. This provides a method to detect objects at wavelengths at which they are transparent.
This seminal work demonstrates that objects can be imaged exclusively with light that they never interacted with. This can be relevant for imaging objects at wavelengths for which efficient detectors do not exist. https://doi.org/10.1038/nature13586
2018: Squeezed light in LIGO
Having successfully detected gravitational waves in 2016, the Laser interferometer Gravitational Wave Observatory (LIGO) upgraded its setup to include squeezed light to enhance sensitivity. Gravitational waves, famously predicted by Einstein, are tiny ripples, many times smaller than a proton, in the fabric of space and time and are the consequence of interacting masses. LIGO splits a high-power laser between two perpendicular arms, 4 km long, where they bounce off mirrors before being recombined and the interference between the two beams is measured. When gravitational waves deform spacetime the lengths of these arms change leading to a change in the interference pattern. LIGO was sensitive enough that vacuum fluctuations were inserting noise into the interferometer, limiting the precision. This upgrade inserted a squeezed vacuum state to supress this noise.
Reference: https://doi.org/10.1103/PhysRevLett.123.231107
This review article offers an overview of squeezing, and specifically its use in laser interferometers. This highlight squeezing in the photon number observable to enhance photocurrent measurements. It touches on the test of squeezing in LIGO in 2013 before its implementation in 2018.
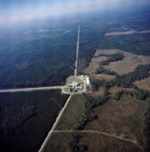
2021: Quantum Advantage in microscopy
The stochastic nature of light poses limits to the achievable microscope sensitivity and resolution when operated at low intensities. However, quantum correlations were shown for the first time to provide a quantum advantage of 35% to the signal to noise ratio over the classical limit.
This paper demonstrates a subshot noise Raman scattering microscope. These are normally shot noise limited so employing squeezing to below the shot noise limit is vital to improving performance without increasing the intensity.
2022: Quantum Gravity Gradient Sensor
A quantum gravity gradient sensor was shown to perform measurements faster than a traditional device in a field test. It was able to locate metre sized features in the gravitational field making gravity cartography a realistic proposal. However, the atom interferometry based setup was shown to operate with a similar uncertainty to traditional devices.
Quantum gravimeters are taken out of the lab for the first time for gravitational cartography. This paper introduces the device and shows it has similar resolution to other devices while being significantly faster.