Experimental Realisations
Introduction
Whilst the theory underpinning quantum mechanics has led to the promise of revolutionary technologies there are many challenges in bringing these to fruition. There has been a large push to develop the hardware required for useful quantum devices, leading to a multitude of candidates for the building blocks of quantum computers - qubits. There is currently no clear winner, with platforms being developed using superconductors, trapped ions and photonics to name a few.
1981: First Quantum Dot
First realised by Ekimov, quantum dots are layers of semiconducting materials and can be thought of as artificial atoms. Quantum dots are particularly useful as single photon sources or qubits where the properties may be designed in manufacturing.
Reference: AI Ekimov, AA Onushchenko, Quantum size effect in three-dimensional microscopic semiconductor crystals. JETP Lett 34, 345–349 (1981)
Overview of how quantum dots are made. A video addressing why these quantum mechanical systems are useful.
Above: An image of three quantum dots from the Quantum Dot Group here at the University of Bristol. The dots pictures are small pillars made of many layers of semiconducting material and are only a few micrometres wide. Quantum dots have a variety of applications, including solar cells and in QLED displays. As quantum dots only emit monochromatic light, they are more efficient than other light sources which require colour filtering. Dots can be fabricated on silicon substrates, which allows for integration into standard silicon circuits.
1997: Quantum Teleportation Demonstrated
Quantum teleportation, with information encoded within the degrees of freedom of photons, was demonstrated for the first time by two groups lead by Popsecu and Zeilinger.
Reference: https://ui.adsabs.harvard.edu/abs/1998PhRvL..80.1121B
A short video by minutephysics detailing how two parties can undertake quantum teleportation.
1998: First Implementation of a quantum algorithms
A two-qubit NMR quantum computer successfully solved Deutsch's problem, marking the first quantum algorithm demonstrated on a physical system. NMR computers, where information is encoded into nuclear spins of atoms, would later be superseded by platforms including superconducting qubits and trapped ions.
Reference: https://doi.org/10.1038/30687
Paper detailing how ensemble computing using NMR spectroscopy is undertaken at the hardware level. An article by Quantiki explaining both what Grover's algorithm is, why it provides speedup and how to run such an algorithm on a quantum computer.
1999: First Superconducting Qubit
The first superconducting qubit was built by Nakamura et al. This was a charge qubit where the basis states are represented by the absence or presence of electron pairs in the Josephson junction.
First realisation of a superconducting charge qubit https://journals.aps.org/prb/abstract/10.1103/PhysRevB.60.15398.
A video by Qiskit addresses the mathematics of classical resonators, before extending to show how superconducting qubits are created by deriving the hamiltonian and corresponding energy spectrum.
Above: An example of a type of Superconducting qubit called a fluxonium qubit. This modern qubit is a derived from the very first superconducting qubit- the charge qubit. Additional components such as inductive elements allow the Fluxonium to preserve the quantum state for much longer than the original charge qubit could. These superconducting qubits are fabricated by depositing thin layers of metal.
2003: All optical Controlled NOT gate
The CNOT gate, a two-qubit entangling gate that is critical for quantum computing, was demonstrated for the first-time using photonics. O’Brien et al. used a probabilistic scheme, similar to that proposed by KLM, to create this gate with a success probability of 1/9, proving the feasibility of photonics as a platform for quantum computing.
O'Brien demonstrate the first all optical CNOT gate using bulk optics https://www.nature.com/articles/nature02054.
CQC2T lecture on how linear optics and projective measurement schemes can be used to create quantum computers. A paper detailing how to create CNOT gates in the coincidence basis.
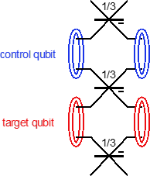
2006: Ion Trap in a Semiconductor Chip
Ionised Cadmium atoms were trapped floating over the surface of a microelectronic chip, by Hensinger et al. Manufactured in the same way as computer chips, they provide a compact and scalable way to build a quantum computer. Wires in the chip generate electric fields which attract or repel the ion to provide spatial confinement, allowing it to be cooled and controlled using lasers. Before this, ions were trapped in bulky traps that were too large to use for a quantum computer.
Reference: https://www.nature.com/articles/nphys171
The University of Oxford Ion trapping department shows the use-cases of ion traps for sensing, computing and quantum information.
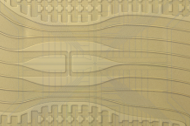
2007: CNOT gate implemented on Superconducting Qubits
Two-qubit controlled-NOT gates were demonstrated on superconducting flux qubits by Plantenberg et al.
Reference: https://pubmed.ncbi.nlm.nih.gov/17568742/
An article showing the analogous behaviour between classical and quantum CNOT gates, with the associated unitary matrices.
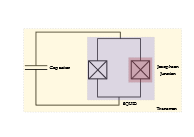
2007: Transmon Qubit Invented
A new type of superconducting qubit with a lower sensitivity to noise than previous designs was invented. The transmon would go on to form the basis of the Google Sycamore processor, which is touted as the first quantum computer to show a quantum advantage.
A theoretical paper detailing the benefits of using flux qubits, whereby the information is contained within the magnetic flux instead of oscillating charge. A video showing the difference between charge qubits and transmon qubits, with an overview of how LC oscillators work.
2009: Shor’s Algorithm on a photonic chip
Researchers at the University of Bristol used photonic qubits to implement Shor’s algorithm to factor fifteen. This was the first quantum algorithm performed on a photonic chip.
Politi, Matthews and O’Brien publish a paper showing their demonstration of Shor’s algorithm on a photonic chip. https://www.science.org/doi/10.1126/science.1173731
Above: A photo of the original photonic chip used to implement Shor’s algorithm at the University of Bristol. Light is guided through the chip in structures called waveguides, the tiny lines you can just make out. As these waveguides are around 1 micrometre wide experiments that would take up a whole lab can be condensed down to a tiny footprint.
2013: Quantum Computer available on the cloud
Bristol reveals an internet-facing two-qubit quantum computing circuit, accessible to the general public.
Press release from Bristol celebrating the launch of the internet-facing 2 qubit optical quantum computer http://www.bris.ac.uk/news/2013/9720.html.
Additional cloud computing services, offered by amazon, enable researchers to connect to a variety of platforms such as superconducting and trapped ion quantum computers.
2015: Two qubit gate in silicon
Two-qubit controlled-NOT gates were demonstrated in silicon. Qubits were formed from silicon spin states and interacted via the spin-spin exchange interaction.
Paper detailing the experimental realisation of the 2 qubit gate in Silicon. This platform holds the record for the highest two-qubit gate fidelities of any currently known qubit: https://www.nature.com/articles/nature15263.
An article addressing scalability issues and CMOS lithographic manufacturing processing of Silicon qubits.
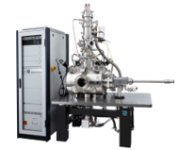
2016: IBM cloud quantum
IBM delivers cloud access to a quantum computer starting with a five-qubit superconducting machine.
Article celebrating the launch of the IBM cloud service, enabling anyone to access a superconducting quantum computer using the circuit based model https://research.ibm.com/blog/quantum-five-years
2019: Boson sampling with 14 photons
Wang et al. demonstrated boson sampling by detecting up to 14 photons from a 60-mode optical interferometer. This demonstrated a significant step towards a quantum advantage.
Reference: https://journals.aps.org/prl/abstract/10.1103/PhysRevLett.123.250503
2019: Quantum Advantage achieved
Google claimed to have achieved quantum advantage for the first time with their 53-qubit superconducting quantum processor - Sycamore. The team sampled the output state from the processor after applying a series of one and two-qubit gates. Whilst it took 200 seconds to get ~106 samples of this distribution, it was theorised to take the best classical algorithm 10,000 years. This claim was disputed by IBM who claimed to have a classical algorithm that could produce samples in only 2.5 days. This was confirmed by subsequent classical algorithms which have enabled faster sampling from the required distribution. Despite this, it represented a significant milestone on the route to competitive quantum technologies.
Google's paper claiming quantum supremacy using their superconducting quantum computer: https://www.nature.com/articles/s41586-019-1666-5.
A short video by Google explaining how their computer outperforms the best classical counerparts. A blog post by IBM refuting the claim that no classical processor can sample from the probability distribution Google used. Article showing how the Theoretical Physics group at the Chinese Academy of Sciences solved the sampling problem in just 15 hours using graphical processing units.
Google used the term Quantum Supremacy when referring to their result. This term has come under much criticism in recent years. Read about it here.
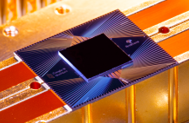
2020: Boson Sampling Achieves a Quantum Advantage
Expanding on previous gaussian boson sampling experiments, Pan’s group detected up to 76 photons in a 100-mode interferometer. They claimed that the best supercomputer would have taken 1014 times longer to generate samples from the same probability distribution. Whilst this marked the first-time boson sampling had been used to demonstrate quantum advantage, there was scrutiny from the surrounding community. Research from The University of Bristol suggested classical algorithms could in reality perform this calculation this in only a few months.
Paper detailing how Pan's group showed the first claim quantum advantage using boson sampling in bulk optics https://www.science.org/doi/10.1126/science.abe8770.
An archive paper detailing what boson sampling is, why it is hard to do classically, and an introduction to complexity theory. A Bristol paper refute the claim from Pan's group, showing that classical computer could perform GBS in just nine months, for nine orders of magnitude over previous experiments.
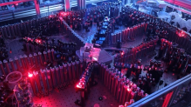
2021: Trapped ion shuttling
Ions were successfully shuttled between two modular chips, a huge step toward a scalable trapped ion quantum computer.
Nature article from Heisinger's group showing how they use microchips and electrodes to shuttle ions: https://media.nature.com/original/magazine-assets/d41586-021-00844-z/d41586-021-00844-z.pdf.
Above: An image of the electronic chips used to shuttle ions at the University of Sussex. Two semiconductor chips for ion trapping are brought together and precisely aligned so that ions can be moved between them. You can see the transfer point at the centre of the gap between the two chips.
2022: Quantum advantage on programmable photonic processor
Xanadu demonstrated quantum advantage through Boson sampling on a programmable photonic quantum processor which was subsequently made available on the cloud. The result detected up to 219 photons across 216 modes.
Xanadu, a company specialising in quantum photonics, released a paper showing how by entangling photons in time, they show quantum advantage when performing sampling using squeezed light: https://www.nature.com/articles/s41586-022-04725-x.